Farming & Agri-Tech: Precision Agriculture Tools for Farmers
Discover how precision agriculture tools are transforming farming with advanced technologies like GPS, drones, IoT sensors, and AI. Learn about their benefits, applications, and future trends to enhance productivity and sustainability.
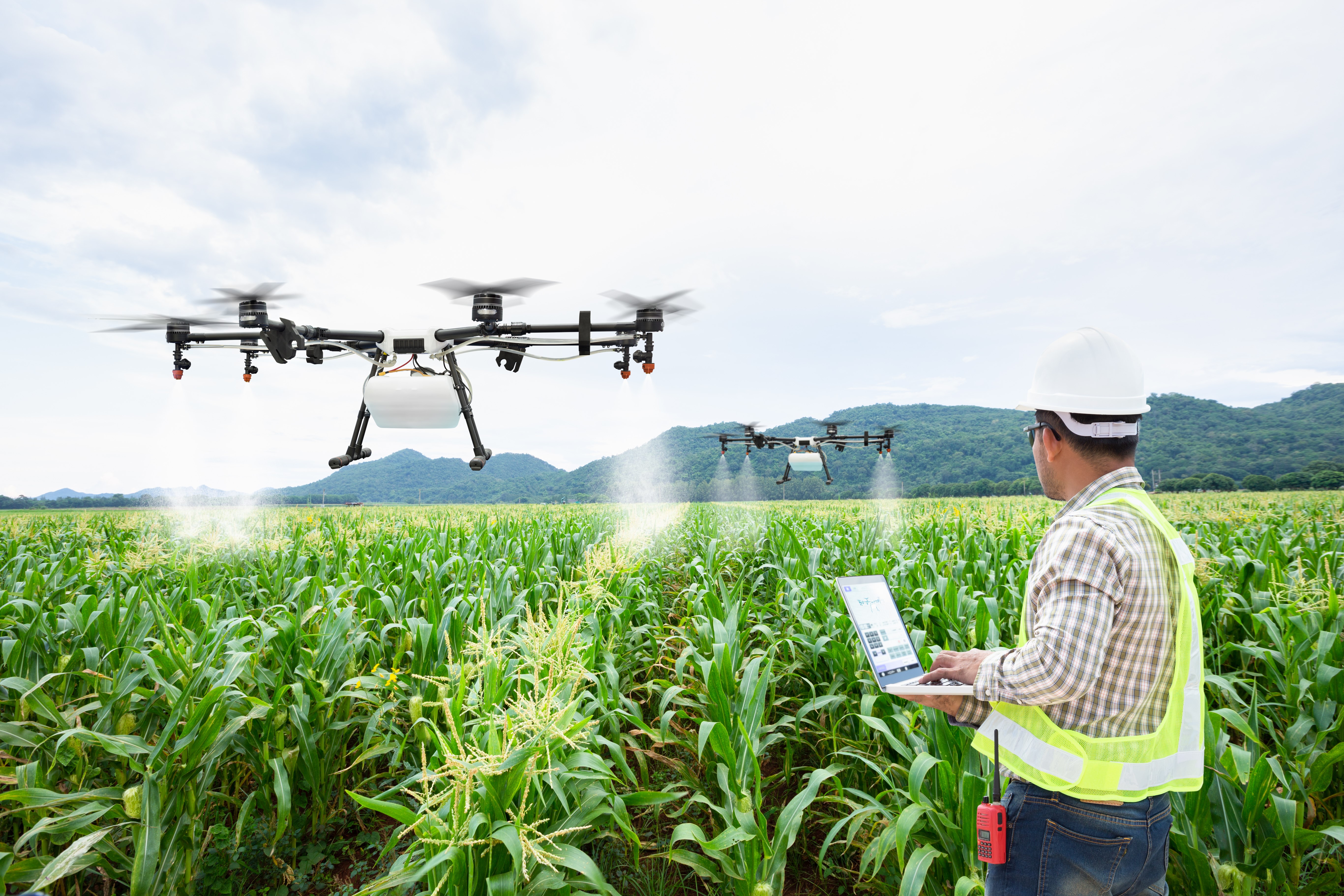
Precision agriculture represents a transformative approach in modern farming, leveraging advanced technologies to optimize agricultural practices. By integrating data-driven insights with innovative tools, farmers can enhance productivity while minimizing resource wastage. The significance of precision agriculture lies in its ability to address global challenges such as food security, environmental sustainability, and economic efficiency. As the global population continues to grow, the demand for food production intensifies, necessitating smarter and more efficient farming methods. Precision agriculture fulfills this need by enabling farmers to make informed decisions based on real-time data.
The adoption of precision agriculture tools is not merely an option but a necessity for modern farmers striving to remain competitive. These tools allow for precise monitoring and management of crop health, soil conditions, water usage, and pest control. By utilizing satellite imagery, sensors, drones, and machine learning algorithms, farmers can achieve unparalleled levels of accuracy in their operations. This technological evolution has redefined traditional farming practices, empowering farmers to maximize yields while reducing input costs. Furthermore, precision agriculture contributes to sustainable farming by minimizing the environmental footprint through optimized resource allocation.
In this article, we delve into the intricacies of precision agriculture tools, exploring their functionalities, benefits, and applications. We aim to provide a comprehensive guide that equips farmers with the knowledge to adopt these cutting-edge technologies effectively. From understanding the role of GPS-guided machinery to harnessing the power of artificial intelligence, this exploration will illuminate how precision agriculture is reshaping the future of farming.
The Evolution of Farming Technology: From Traditional Practices to Modern Innovations
The journey of farming technology has been one of continuous evolution, transitioning from rudimentary tools to sophisticated systems that define modern agriculture. In the early days of agriculture, farmers relied heavily on manual labor and basic implements such as plows and sickles. These tools, though effective for their time, were labor-intensive and limited in scope, often resulting in inconsistent yields. The introduction of animal-drawn equipment marked the first significant leap forward, allowing farmers to cultivate larger areas with greater efficiency. However, the reliance on animals also brought challenges, including maintenance costs and limitations in speed and precision.
The Industrial Revolution ushered in a new era of mechanization, transforming agriculture with the advent of steam-powered machinery and later, internal combustion engines. Tractors, harvesters, and other motorized equipment revolutionized farming by drastically reducing the time and effort required for planting, cultivating, and harvesting crops. These innovations not only increased productivity but also enabled farmers to manage larger farms with fewer workers. Despite these advancements, traditional farming methods remained largely reactive, with decisions based on experience and intuition rather than data-driven insights.
The late 20th century witnessed the emergence of digital technologies, which laid the foundation for precision agriculture. The integration of computers, sensors, and satellite systems introduced unprecedented levels of accuracy and control in farming operations. Global Positioning Systems (GPS) became instrumental in guiding machinery with pinpoint accuracy, reducing overlap and ensuring uniform application of inputs such as fertilizers and pesticides. Simultaneously, Geographic Information Systems (GIS) provided farmers with detailed maps of their fields, enabling them to analyze spatial variations in soil properties, moisture levels, and crop health.
As we entered the 21st century, the convergence of big data, artificial intelligence, and the Internet of Things (IoT) further propelled farming technology into uncharted territory. Drones equipped with multispectral cameras now offer aerial insights into crop conditions, while IoT-enabled sensors monitor soil moisture, temperature, and nutrient levels in real-time. Machine learning algorithms process vast datasets to predict pest outbreaks, optimize irrigation schedules, and recommend tailored interventions. These innovations have shifted agriculture from a generalized approach to a highly customized and data-centric model, where every decision is informed by precise measurements and predictive analytics.
This evolution underscores the profound impact of technology on farming practices, highlighting how each advancement has built upon the last to create a more efficient, sustainable, and productive agricultural system. As we continue to embrace agri-tech solutions, the potential for further innovation remains limitless, promising a future where farming is not only smarter but also more resilient in the face of global challenges.
Understanding Precision Agriculture Tools: A Comprehensive Overview
Precision agriculture tools encompass a diverse array of technologies designed to enhance farming efficiency and sustainability. At the core of these tools are Global Positioning Systems (GPS) , which provide farmers with accurate geospatial data to guide machinery and optimize field operations. GPS technology enables the creation of detailed field maps, allowing for precise tracking of crop growth patterns, soil variability, and resource distribution. This level of accuracy ensures that inputs such as seeds, fertilizers, and pesticides are applied uniformly, reducing waste and improving yield outcomes.
Complementing GPS are Geographic Information Systems (GIS) , which serve as powerful platforms for analyzing and visualizing spatial data. GIS software integrates multiple layers of information, such as topography, soil types, and historical yield records, to generate actionable insights. Farmers can use GIS to identify high-performing zones within their fields, prioritize resource allocation, and implement targeted interventions. For instance, GIS mapping can reveal areas prone to waterlogging or nutrient deficiencies, enabling proactive measures to mitigate these issues.
Another cornerstone of precision agriculture is the use of drones equipped with advanced imaging capabilities. These unmanned aerial vehicles (UAVs) capture high-resolution images and multispectral data, offering a bird's-eye view of crop health and field conditions. Drones are particularly effective in detecting early signs of stress, such as pest infestations or nutrient imbalances, long before they become visible to the naked eye. The data collected by drones can be processed using specialized software to generate vegetation indices, such as the Normalized Difference Vegetation Index (NDVI), which quantify plant vigor and biomass.
On the ground, IoT-enabled sensors play a critical role in monitoring real-time environmental parameters. These sensors are deployed across fields to measure variables such as soil moisture, temperature, pH levels, and nutrient concentrations. By transmitting data wirelessly to centralized platforms, IoT sensors enable farmers to make timely adjustments to irrigation schedules, fertilizer applications, and pest management strategies. For example, soil moisture sensors can trigger automated irrigation systems when predefined thresholds are reached, ensuring optimal water usage and preventing over-irrigation.
Machine learning and artificial intelligence (AI) further amplify the capabilities of precision agriculture tools by enabling predictive analytics and decision support. AI algorithms analyze vast datasets from multiple sources, including satellite imagery, weather forecasts, and historical farm records, to forecast trends and recommend actions. Predictive models can anticipate pest outbreaks, disease spread, and adverse weather events, empowering farmers to take preemptive measures. Additionally, AI-driven platforms can simulate various scenarios, helping farmers evaluate the potential outcomes of different management strategies before implementation.
Together, these tools form an interconnected ecosystem that transforms raw data into actionable intelligence. By leveraging GPS, GIS, drones, IoT sensors, and AI, farmers gain unparalleled visibility into their operations, enabling them to optimize resource utilization, reduce environmental impact, and achieve higher productivity. This integrated approach not only enhances the efficiency of individual tasks but also fosters a holistic understanding of the entire farming process, paving the way for sustainable and resilient agricultural practices.
The Role of GPS-Guided Machinery in Precision Agriculture
GPS-guided machinery stands at the forefront of precision agriculture, revolutionizing the way farmers interact with their fields. This technology utilizes satellite-based positioning systems to provide real-time location data, enabling machinery to operate with unmatched precision. Tractors, harvesters, and other farm equipment equipped with GPS receivers can follow pre-programmed paths with centimeter-level accuracy, significantly reducing overlap and ensuring consistent coverage. This level of precision minimizes the wastage of seeds, fertilizers, and pesticides, which are often applied unevenly in traditional farming practices.
One of the most impactful applications of GPS-guided machinery is in variable rate technology (VRT) . VRT allows farmers to adjust the application rates of inputs such as fertilizers, herbicides, and water based on the specific needs of different areas within a field. By integrating GPS data with soil maps and yield monitors, farmers can create prescription maps that dictate the exact amount of input required for each section of the field. For example, areas with higher soil fertility may receive less fertilizer, while nutrient-deficient zones are treated more intensively. This targeted approach not only optimizes resource usage but also reduces the risk of over-application, which can lead to environmental degradation.
Moreover, GPS-guided machinery facilitates automated steering systems , which alleviate the physical and mental strain on operators. Automated steering eliminates human error, ensuring that machinery follows the intended path without deviation. This capability is particularly valuable during repetitive tasks such as planting and spraying, where fatigue can compromise accuracy. Additionally, automated steering systems enable nighttime operations, extending the working hours available for critical tasks like harvesting.
The integration of GPS with telematics systems further enhances the functionality of farm machinery. Telematics platforms collect and transmit data on machine performance, fuel consumption, and operational efficiency, providing farmers with valuable insights into their equipment's usage. This data can be analyzed to optimize maintenance schedules, reduce downtime, and improve overall fleet management. For instance, telematics can alert farmers to potential mechanical issues before they escalate, ensuring that machinery remains in peak condition throughout the growing season.
Beyond operational efficiency, GPS-guided machinery contributes to environmental sustainability by minimizing the ecological footprint of farming activities. Precise application of inputs reduces runoff and leaching, which can contaminate nearby water bodies. Similarly, optimized fuel consumption lowers greenhouse gas emissions, aligning farming practices with global efforts to combat climate change. By adopting GPS-guided machinery, farmers not only enhance their productivity but also demonstrate a commitment to responsible stewardship of natural resources.
In summary, GPS-guided machinery is a cornerstone of precision agriculture, offering unparalleled accuracy and efficiency in field operations. Its ability to integrate with other technologies, such as variable rate application and telematics, amplifies its impact, enabling farmers to achieve optimal results while promoting sustainability. As this technology continues to evolve, its role in shaping the future of agriculture becomes increasingly indispensable.
Drones in Agriculture: Enhancing Crop Monitoring and Management
Drones have emerged as a transformative tool in precision agriculture, offering farmers unprecedented capabilities in crop monitoring and management. Equipped with advanced imaging technologies, these unmanned aerial vehicles (UAVs) provide high-resolution aerial views of farmland, enabling detailed assessments of crop health and field conditions. One of the primary advantages of drones is their ability to capture multispectral and thermal imagery , which goes beyond the visible spectrum to reveal hidden stressors affecting plants. For instance, multispectral cameras can detect variations in chlorophyll content, identifying areas of nutrient deficiency or water stress that are invisible to the naked eye.
The data collected by drones is instrumental in generating vegetation indices , such as the Normalized Difference Vegetation Index (NDVI), which quantifies plant vigor and biomass. NDVI maps highlight regions of the field where crops are thriving or struggling, allowing farmers to target interventions with remarkable precision. For example, areas showing low NDVI values may indicate pest infestations or disease outbreaks, prompting immediate action to mitigate damage. This proactive approach not only prevents yield losses but also reduces the need for blanket applications of pesticides, promoting environmentally friendly farming practices.
In addition to monitoring crop health, drones play a crucial role in field mapping and surveying . High-resolution orthomosaic maps created from drone imagery provide farmers with accurate representations of their fields, complete with elevation data and boundary delineations. These maps are invaluable for planning irrigation systems, designing drainage networks, and optimizing land use. Moreover, drones can quickly survey large areas, making them ideal for assessing post-harvest residue, evaluating soil erosion, and monitoring changes in land cover over time.
Drones also excel in pest and disease management , offering rapid detection and response capabilities. Thermal imaging sensors can identify hotspots of pest activity or fungal infections, enabling targeted treatments that minimize chemical usage. For instance, drones equipped with spray systems can deliver precise doses of biopesticides or fungicides directly to affected areas, reducing the environmental impact while ensuring effective control. This level of precision is particularly beneficial in organic farming, where chemical inputs are limited, and alternative solutions are essential.
Furthermore, drones contribute to water management by assessing irrigation efficiency and identifying areas of water stress. Thermal cameras can detect variations in canopy temperature, which correlate with moisture levels in the soil. By pinpointing dry zones or over-irrigated areas, farmers can adjust their irrigation strategies to optimize water usage and conserve this vital resource. This capability is especially critical in regions facing water scarcity, where efficient irrigation practices are paramount for sustainable agriculture.
The versatility of drones extends to crop scouting and inventory management as well. Farmers can deploy drones to conduct routine inspections of fields, identifying weeds, lodging, or other anomalies that require attention. During harvest, drones can estimate crop yields by analyzing plant density and maturity levels, providing valuable insights for logistical planning. Additionally, drones facilitate the creation of digital inventories by capturing detailed images of stored produce, ensuring accurate record-keeping and traceability.
By integrating drones into their operations, farmers gain access to real-time, actionable data that enhances decision-making and improves overall farm efficiency. The ability to monitor crops comprehensively, detect issues early, and implement targeted interventions positions drones as an indispensable tool in modern agriculture. As drone technology continues to advance, its applications in precision agriculture are expected to expand, further solidifying its role in driving sustainable and productive farming practices.
IoT Sensors in Agriculture: Real-Time Data Collection and Analysis
The integration of Internet of Things (IoT) sensors in agriculture has revolutionized the way farmers gather and utilize data to optimize their operations. These sensors, strategically placed across fields, provide real-time insights into critical environmental parameters, enabling farmers to make informed decisions that enhance productivity and sustainability. At the heart of this transformation is the ability of IoT sensors to continuously monitor variables such as soil moisture, temperature, humidity, and nutrient levels, delivering precise and actionable data to centralized platforms.
One of the most impactful applications of IoT sensors is in soil health monitoring . Soil moisture sensors, for instance, measure the water content in the soil at various depths, allowing farmers to tailor irrigation schedules to the specific needs of their crops. This ensures that plants receive adequate hydration without overwatering, which can lead to root rot or nutrient leaching. Similarly, sensors that track soil temperature and pH levels help farmers assess the suitability of their fields for different crops, guiding decisions on crop rotation and planting schedules. By maintaining optimal soil conditions, farmers can maximize yield potential while conserving water and reducing input costs.
IoT sensors also play a pivotal role in climate-smart agriculture by providing real-time weather data directly from the field. Temperature and humidity sensors, combined with wind speed and rainfall gauges, offer localized weather insights that are more accurate than regional forecasts. This granular data enables farmers to anticipate adverse weather events, such as frost or heatwaves, and take preventive measures to protect their crops. For example, automated systems triggered by sensor data can deploy frost protection mechanisms or adjust greenhouse ventilation to maintain ideal growing conditions.
Another significant advantage of IoT sensors is their ability to facilitate nutrient management . Nitrogen, phosphorus, and potassium sensors measure the availability of essential nutrients in the soil, enabling farmers to apply fertilizers with precision. This targeted approach minimizes the risk of over-fertilization, which can lead to environmental pollution, while ensuring that crops receive the nutrients they need for optimal growth. Additionally, sensors that monitor leaf wetness and canopy temperature help farmers identify early signs of nutrient deficiencies or stress, allowing for timely corrective actions.
The data collected by IoT sensors is transmitted wirelessly to cloud-based platforms, where it is analyzed using advanced algorithms to generate actionable insights. Farmers can access these insights through user-friendly dashboards on smartphones or tablets, enabling remote monitoring and decision-making. For instance, if a sensor detects a sudden drop in soil moisture levels, the system can send an alert to the farmer, who can then activate an irrigation system remotely. This seamless integration of hardware and software streamlines farm operations, saving time and labor while improving efficiency.
Furthermore, IoT sensors contribute to predictive analytics by feeding large datasets into machine learning models. These models analyze historical and real-time data to forecast trends, such as pest outbreaks or disease spread, empowering farmers to take preemptive measures. For example, a combination of soil moisture, temperature, and humidity data can predict the likelihood of fungal infections, prompting farmers to apply protective treatments before symptoms appear. This proactive approach not only safeguards crop health but also reduces reliance on reactive measures, which are often costlier and less effective.
The scalability of IoT sensor networks makes them suitable for farms of all sizes, from smallholder operations to large-scale commercial enterprises. Modular designs allow farmers to start with a few sensors and expand their network as needed, ensuring a cost-effective and flexible solution. Additionally, the interoperability of IoT systems enables seamless integration with other precision agriculture tools, such as GPS-guided machinery and drones, creating a cohesive ecosystem that maximizes the value of collected data.
In summary, IoT sensors represent a cornerstone of modern agriculture, providing farmers with real-time data that drives efficiency, sustainability, and resilience. By enabling precise monitoring and management of environmental parameters, these sensors empower farmers to optimize resource usage, mitigate risks, and achieve higher yields. As IoT technology continues to evolve, its role in shaping the future of agriculture will only grow, offering new opportunities for innovation and progress.
Artificial Intelligence and Machine Learning: Revolutionizing Agricultural Decision-Making
Artificial intelligence (AI) and machine learning (ML) have emerged as transformative forces in agriculture, offering farmers unprecedented capabilities in data analysis, prediction, and decision-making. These technologies leverage vast datasets generated by IoT sensors, drones, satellites, and historical farm records to uncover patterns and insights that would be impossible to discern manually. By applying advanced algorithms, AI and ML systems can process this data to provide actionable recommendations, enabling farmers to make informed choices that enhance productivity and sustainability.
One of the most significant applications of AI in agriculture is predictive modeling . Machine learning algorithms analyze historical weather patterns, soil conditions, and crop performance to forecast future outcomes, such as yield potential, pest outbreaks, and disease spread. For instance, predictive models can identify the likelihood of a fungal infection based on factors like temperature, humidity, and leaf wetness, allowing farmers to apply protective treatments before symptoms manifest. This proactive approach not only minimizes crop losses but also reduces the need for reactive measures, which are often more costly and less effective.
AI-driven platforms also excel in yield optimization by simulating various scenarios and recommending tailored interventions. For example, these systems can evaluate the impact of different irrigation schedules, fertilizer application rates, and planting densities on crop yields. By running simulations, farmers can identify the most effective strategies for maximizing productivity while minimizing resource usage. This capability is particularly valuable in regions with limited water or nutrient availability, where efficient resource allocation is critical for sustainable farming.
Another groundbreaking application of AI is in pest and disease management . Machine learning models trained on extensive datasets of pest behavior and disease symptoms can detect early warning signs with remarkable accuracy. For instance, image recognition algorithms can analyze drone-captured imagery to identify pest infestations or disease lesions on leaves, even before they are visible to the human eye. Once detected, AI systems can recommend targeted interventions, such as the application of biopesticides or fungicides, ensuring precise and timely control. This level of precision not only protects crops but also minimizes the environmental impact of chemical treatments.
AI and ML also play a crucial role in resource optimization , particularly in irrigation and fertilization. By analyzing real-time data from IoT sensors, AI systems can determine the exact water and nutrient requirements of crops at different growth stages. For example, machine learning algorithms can adjust irrigation schedules based on soil moisture levels, weather forecasts, and evapotranspiration rates, ensuring optimal hydration while conserving water. Similarly, AI-driven nutrient management systems can recommend precise fertilizer application rates based on soil nutrient profiles and crop demands, reducing wastage and environmental contamination.
The integration of AI with robotics further amplifies its impact on agriculture. Autonomous robots equipped with AI capabilities can perform tasks such as planting, weeding, and harvesting with unparalleled efficiency. For instance, AI-powered weed detection systems can differentiate between crops and weeds, enabling robotic arms to selectively remove unwanted plants without damaging the main crop. This precision not only reduces labor costs but also minimizes the use of herbicides, promoting environmentally friendly farming practices.
Moreover, AI and ML contribute to supply chain optimization by enhancing traceability and inventory management. Predictive analytics can forecast market demand, enabling farmers to align their production schedules with consumer needs. Additionally, AI systems can track the movement of produce from farm to market, ensuring compliance with quality standards and reducing post-harvest losses. This end-to-end visibility not only improves profitability but also strengthens the resilience of agricultural supply chains.
The scalability and adaptability of AI and ML systems make them suitable for farms of all sizes and types. Cloud-based platforms allow farmers to access these technologies without significant upfront investments, democratizing the benefits of advanced analytics. Furthermore, the continuous learning capabilities of machine learning models ensure that these systems improve over time, adapting to changing conditions and delivering increasingly accurate insights.
In conclusion, artificial intelligence and machine learning are reshaping the agricultural landscape by empowering farmers with data-driven tools that enhance decision-making and operational efficiency. From predictive modeling and yield optimization to pest management and resource conservation, these technologies offer a wide range of applications that drive productivity, sustainability, and resilience. As AI and ML continue to evolve, their role in advancing precision agriculture will only grow, unlocking new possibilities for innovation and progress in the farming sector.
Benefits of Adopting Precision Agriculture Tools: Enhanced Efficiency and Sustainability
The adoption of precision agriculture tools offers a multitude of benefits that extend beyond mere technological advancement, fundamentally transforming the way farmers approach their operations. One of the most significant advantages is the substantial improvement in efficiency . Precision agriculture tools enable farmers to allocate resources such as water, fertilizers, and pesticides with pinpoint accuracy, eliminating unnecessary waste and ensuring that every input contributes directly to crop health and yield. For instance, variable rate technology (VRT) allows for the precise application of fertilizers based on soil nutrient maps, ensuring that only the required amount is used in each section of the field. This targeted approach not only reduces input costs but also minimizes the environmental impact, fostering a more sustainable farming ecosystem.
Another critical benefit is the enhancement of productivity . By leveraging real-time data from IoT sensors, drones, and satellite imagery, farmers can identify and address issues such as pest infestations, nutrient deficiencies, and water stress at their earliest stages. Early detection and intervention prevent minor problems from escalating into major threats, safeguarding crop yields and ensuring consistent output. For example, drones equipped with multispectral cameras can detect subtle changes in plant health that are invisible to the naked eye, allowing farmers to take corrective actions before visible symptoms appear. This proactive approach not only maximizes yield potential but also stabilizes income streams, providing farmers with greater financial security.
Sustainability is another cornerstone of precision agriculture, as these tools are designed to minimize the ecological footprint of farming activities. By optimizing resource usage, precision agriculture reduces the over-application of chemicals and water, which are common contributors to environmental degradation. For instance, soil moisture sensors integrated with automated irrigation systems ensure that water is applied only when and where it is needed, conserving this vital resource and preventing runoff that can contaminate nearby water bodies. Similarly, the precise application of fertilizers and pesticides minimizes nutrient leaching and chemical residues, protecting soil health and biodiversity. These practices align with global efforts to combat climate change and promote environmentally responsible farming.
Precision agriculture tools also contribute to cost savings , a benefit that resonates deeply with farmers operating on tight margins. The ability to monitor and manage resources with precision reduces input costs, while predictive analytics and decision-support systems minimize the risk of crop failures. For example, machine learning models that forecast pest outbreaks or disease spread enable farmers to implement preventive measures, avoiding the higher costs associated with reactive treatments. Additionally, the automation of repetitive tasks, such as planting and spraying, reduces labor requirements, freeing up resources for other critical activities. These cost-saving measures not only improve profitability but also enhance the competitiveness of farms in an increasingly challenging market.
Another notable advantage is the improvement in traceability and compliance . Precision agriculture tools generate detailed records of all farming activities, from planting and irrigation to harvesting and post-harvest handling. This level of documentation is invaluable for meeting regulatory requirements and ensuring compliance with food safety standards. For instance, blockchain-enabled platforms can track the movement of produce from farm to market, providing transparent and tamper-proof records that build trust with consumers. Enhanced traceability also facilitates better inventory management, reducing post-harvest losses and ensuring that produce reaches the market at its peak quality.
Finally, precision agriculture tools empower farmers with data-driven insights that enhance decision-making and strategic planning. Advanced analytics platforms process vast datasets to uncover trends and correlations, enabling farmers to make informed choices about crop selection, planting schedules, and resource allocation. For example, predictive models that analyze historical yield data and weather patterns can recommend optimal planting windows, maximizing productivity while minimizing risks. This data-centric approach not only improves short-term outcomes but also supports long-term sustainability by fostering resilience against climate variability and market fluctuations.
In summary, the adoption of precision agriculture tools delivers a wide array of benefits that collectively enhance efficiency, productivity, sustainability, and profitability. By enabling precise resource management, early problem detection, and data-driven decision-making, these tools empower farmers to overcome traditional challenges and embrace a future of smart, resilient, and environmentally conscious farming practices.
Challenges and Limitations of Precision Agriculture Tools: Addressing Barriers to Adoption
Despite the transformative potential of precision agriculture tools, their widespread adoption faces several challenges and limitations that must be addressed to unlock their full benefits. One of the most significant barriers is the high initial investment required to acquire and implement these technologies. Precision agriculture tools, such as GPS-guided machinery, drones, IoT sensors, and AI-driven platforms, often come with substantial upfront costs that can be prohibitive for smallholder farmers or those operating on tight budgets. Even when financing options are available, the perceived financial risk can deter farmers from investing in these tools, particularly in regions where access to credit is limited or interest rates are high. To overcome this challenge, governments and private organizations must explore innovative funding models, such as subsidies, grants, or leasing programs, to make these technologies more accessible and affordable.
Another critical limitation is the technical complexity associated with precision agriculture tools. Many farmers, particularly those in developing regions, lack the technical expertise needed to operate and maintain these advanced systems. For instance, interpreting data from IoT sensors or configuring machine learning models requires specialized knowledge that may not be readily available. This knowledge gap can lead to underutilization of the tools or incorrect implementation, undermining their effectiveness. To address this issue, comprehensive training programs and capacity-building initiatives are essential. Collaborations between agricultural extension services, technology providers, and educational institutions can equip farmers with the skills needed to harness the full potential of precision agriculture tools. Additionally, user-friendly interfaces and intuitive design features can simplify the adoption process, making these technologies more approachable for users with varying levels of technical proficiency.
Data management and cybersecurity concerns also pose significant challenges in the adoption of precision agriculture tools. The vast amounts of data generated by IoT sensors, drones, and satellite systems require robust storage, processing, and analysis capabilities. Farmers often lack the infrastructure needed to handle this data effectively, leading to inefficiencies or incomplete insights. Furthermore, the increasing reliance on digital platforms raises concerns about data privacy and security. Unauthorized access to sensitive farm data, such as yield records or financial information, can have serious consequences for farmers. To mitigate these risks, stakeholders must invest in secure cloud-based platforms and encryption technologies that protect data integrity while ensuring seamless accessibility. Clear policies and regulations governing data ownership and usage are also necessary to build trust among farmers and encourage wider adoption.
The scalability of precision agriculture tools presents another hurdle, particularly for small-scale farmers. While these technologies are highly effective on large commercial farms, their applicability to smaller operations is often limited by cost constraints and logistical challenges. For example, drones and IoT sensor networks may not be practical for farms with fragmented landholdings or limited connectivity. To address this issue, modular and scalable solutions are needed, allowing farmers to start with a minimal setup and expand as their needs grow. Additionally, community-based approaches, such as shared drone services or cooperative IoT networks, can make these tools more accessible to smallholders while fostering collaboration and resource sharing.
Environmental and infrastructure limitations further complicate the adoption of precision agriculture tools. In remote or rural areas, inadequate internet connectivity and unreliable power supply can hinder the deployment of IoT devices and cloud-based platforms. Similarly, harsh environmental conditions, such as extreme temperatures or heavy rainfall, can affect the performance and durability of sensors and machinery. To overcome these challenges, technology providers must develop ruggedized equipment capable of withstanding adverse conditions. Investments in rural infrastructure, such as broadband expansion and renewable energy solutions, are also critical to creating an enabling environment for precision agriculture.
Finally, the cultural and behavioral resistance to change poses a significant barrier to adoption. Many farmers are accustomed to traditional practices and may be hesitant to embrace new technologies, perceiving them as unnecessary or overly complex. Overcoming this resistance requires targeted awareness campaigns and demonstration projects that showcase the tangible benefits of precision agriculture tools. Success stories and testimonials from early adopters can serve as powerful motivators, encouraging others to follow suit. Additionally, involving farmers in the development and testing of new tools can foster a sense of ownership and increase acceptance.
In conclusion, while precision agriculture tools hold immense promise for revolutionizing farming practices, addressing the challenges and limitations outlined above is crucial for their successful adoption. By tackling financial, technical, and infrastructural barriers, and fostering a culture of innovation and collaboration, stakeholders can pave the way for a more inclusive and sustainable agricultural future.
Case Studies: Successful Implementation of Precision Agriculture Tools Across Farms
To illustrate the transformative impact of precision agriculture tools, we examine several case studies from diverse farming operations around the world. These examples highlight how farmers have successfully implemented advanced technologies to overcome challenges, optimize resource usage, and achieve remarkable improvements in productivity and sustainability.
Case Study 1: Large-Scale Corn Farm in the United States
A 5,000-acre corn farm in Iowa adopted GPS-guided machinery and variable rate technology (VRT) to address inconsistencies in soil fertility and water retention. Prior to implementation, the farm faced challenges with over-application of fertilizers and uneven crop yields due to soil variability. By integrating soil maps generated through GIS with GPS-enabled tractors, the farm implemented a VRT system that adjusted fertilizer application rates based on specific soil nutrient profiles. The results were striking: fertilizer usage decreased by 20%, while corn yields increased by 15%. Additionally, the farm reported a 30% reduction in fuel consumption due to optimized machinery paths, demonstrating both economic and environmental benefits.
Case Study 2: Vineyard in France Utilizing Drones and IoT Sensors
A vineyard in Bordeaux leveraged drones and IoT sensors to enhance grape quality and streamline pest management. The vineyard faced recurring issues with fungal diseases and inconsistent grape ripening, which affected wine quality. Drones equipped with multispectral cameras were deployed weekly to monitor vine health, generating NDVI maps that highlighted stressed areas. Simultaneously, IoT soil moisture sensors provided real-time data on irrigation needs, enabling precise water delivery. The integration of these tools allowed the vineyard to detect and treat fungal infections early, reducing pesticide usage by 40%. Grape uniformity improved significantly, leading to a 25% increase in premium-grade harvests and higher market prices for the wine produced.
Case Study 3: Smallholder Rice Farm in India Using AI-Powered Platforms
A cooperative of smallholder rice farmers in Punjab adopted an AI-driven platform to optimize irrigation and nutrient management. Water scarcity and inefficient fertilizer use were major challenges for the farmers, who relied on traditional flood irrigation methods. The AI platform analyzed data from weather forecasts, soil sensors, and historical yield records to recommend precise irrigation schedules and fertilizer application rates. Farmers accessed these recommendations via a mobile app, which also provided alerts for pest outbreaks. Within two seasons, water usage decreased by 35%, fertilizer costs dropped by 25%, and rice yields increased by 20%. The cooperative reported a collective income boost of 18%, demonstrating the scalability of AI solutions for small-scale operations.
Case Study 4: Organic Vegetable Farm in Australia Leveraging Machine Learning
An organic vegetable farm in Victoria implemented machine learning algorithms to enhance pest control and crop rotation planning. The farm struggled with pest infestations and declining soil health due to suboptimal crop rotations. Machine learning models analyzed data from past growing seasons, including pest behavior, soil nutrient levels, and crop performance, to recommend optimal rotation sequences. The models also predicted pest outbreaks based on weather patterns and field conditions, enabling preemptive biological controls. As a result, pest-related crop losses decreased by 50%, and soil organic matter increased by 15%. The farm achieved a 12% rise in overall yield while maintaining its organic certification, underscoring the compatibility of precision agriculture with sustainable practices.
Case Study 5: Dairy Farm in New Zealand Using IoT and Robotics
A dairy farm in Canterbury integrated IoT sensors and autonomous robots to improve pasture management and milk production. The farm faced challenges with uneven grazing patterns and labor shortages during peak milking periods. IoT sensors monitored pasture growth and soil moisture, providing data to optimize rotational grazing schedules. Autonomous robots handled tasks such as herding and milking, reducing labor requirements by 40%. The precision in pasture management led to a 10% increase in grass utilization, while milk production rose by 8%. The farm also reported a 20% reduction in operational costs, highlighting the dual benefits of efficiency and profitability.
These case studies underscore the versatility and effectiveness of precision agriculture tools across different farming contexts. Whether addressing resource inefficiencies, enhancing crop quality, or overcoming labor constraints, these technologies have consistently delivered measurable improvements. By tailoring solutions to specific challenges and leveraging data-driven insights, farmers worldwide are achieving new levels of productivity and sustainability, setting benchmarks for the future of agriculture.
Future Trends in Precision Agriculture: Emerging Technologies and Innovations
The future of precision agriculture is poised to be shaped by a wave of emerging technologies and innovations that promise to further enhance farming efficiency, sustainability, and resilience. Among these advancements, blockchain technology stands out as a transformative force with the potential to revolutionize supply chain transparency and traceability. By creating immutable, decentralized ledgers, blockchain can track the journey of agricultural products from farm to market, ensuring authenticity and compliance with food safety standards. For instance, blockchain-enabled platforms can verify organic certifications, monitor cold chain logistics, and provide consumers with detailed information about the origin and handling of their food. This level of transparency not only builds trust but also opens new opportunities for premium pricing and market differentiation.
Another groundbreaking trend is the integration of 5G connectivity into agricultural operations. The ultra-fast and reliable communication capabilities of 5G networks will enable real-time data transmission from IoT sensors, drones, and autonomous machinery, facilitating seamless coordination across farm activities. For example, 5G-powered drones can stream high-resolution imagery to cloud-based platforms for instant analysis, allowing farmers to respond to crop stressors within minutes. Similarly, autonomous tractors and harvesters connected via 5G can operate in synchronized fleets, optimizing field coverage and reducing overlap. This enhanced connectivity will also support edge computing, where data processing occurs closer to the source, reducing latency and improving decision-making speed.
Autonomous robots are set to play an increasingly prominent role in precision agriculture, performing tasks ranging from planting and weeding to harvesting and post-harvest handling. Advances in robotics, coupled with AI and computer vision, are enabling robots to navigate complex terrains, identify crops and weeds with precision, and execute delicate operations such as fruit picking without damaging produce. For instance, robotic weeders equipped with AI-powered cameras can distinguish between crops and invasive plants, applying targeted treatments or mechanical removal. These robots not only reduce labor dependency but also minimize chemical usage, aligning with sustainable farming goals.
The convergence of biotechnology and precision agriculture is another exciting frontier. CRISPR gene-editing technology, for example, can be used to develop crop varieties with enhanced resistance to pests, diseases, and environmental stresses. When combined with precision agriculture tools, such as drones and IoT sensors, these genetically optimized crops can be monitored and managed with unprecedented precision. For instance, sensors can detect early signs of stress in drought-resistant crops, triggering automated irrigation systems to maintain optimal hydration levels. This synergy between biotechnology and precision agriculture holds the potential to significantly boost yields while reducing resource inputs.
Augmented reality (AR) and virtual reality (VR) are emerging as powerful tools for training and simulation in agriculture. AR applications can overlay real-time data onto physical fields, providing farmers with interactive insights into soil conditions, crop health, and machinery performance. VR, on the other hand, can simulate various farming scenarios, enabling farmers to test strategies and visualize outcomes before implementation. For example, VR platforms can model the impact of different irrigation techniques or pest control measures, helping farmers make informed decisions. These immersive technologies not only enhance learning but also foster innovation by allowing farmers to experiment in a risk-free environment.
Finally, the rise of digital twins in agriculture is set to transform farm management by creating virtual replicas of physical fields and operations. Digital twins integrate data from multiple sources, such as IoT sensors, drones, and weather forecasts, to simulate real-world conditions and predict outcomes. Farmers can use these virtual models to optimize resource allocation, plan crop rotations, and anticipate challenges such as pest outbreaks or weather extremes. For instance, a digital twin of a vineyard can simulate the effects of different pruning techniques on grape quality, guiding decisions that maximize yield and market value. This predictive capability empowers farmers to proactively address issues and capitalize on opportunities, driving efficiency and sustainability.
In conclusion, the future of precision agriculture is characterized by a convergence of cutting-edge technologies that amplify its impact and expand its applications. From blockchain and 5G to autonomous robots and biotechnology, these innovations are set to redefine the boundaries of what is possible in farming. By embracing these trends, farmers can position themselves at the forefront of a new era in agriculture, marked by unprecedented levels of productivity, sustainability, and resilience.
Accessibility: Making Precision Agriculture Tools Available to All Farmers
Ensuring the accessibility of precision agriculture tools is a critical step toward democratizing advanced farming technologies and empowering farmers of all scales to participate in the digital agricultural revolution. Accessibility begins with addressing the financial barriers that often prevent smallholder and resource-constrained farmers from adopting these tools. Governments, non-governmental organizations (NGOs), and private companies must collaborate to develop innovative financing models, such as low-interest loans, leasing programs, and subsidy schemes, to make precision agriculture tools more affordable. For instance, public-private partnerships can fund community-based initiatives where groups of smallholder farmers collectively invest in shared resources like drones or IoT sensor networks, spreading costs and maximizing utility.
Equally important is the need to bridge the digital divide that limits access to precision agriculture tools in rural and remote areas. Expanding broadband internet infrastructure and ensuring reliable connectivity are foundational steps to enabling the use of cloud-based platforms, IoT devices, and real-time data analytics. Governments and telecommunications companies should prioritize investments in rural broadband expansion to ensure that even the most isolated farming communities can access these technologies. Additionally, the development of offline-capable solutions can serve as a temporary bridge for areas where internet connectivity remains unreliable. For example, mobile applications designed for precision agriculture can store data locally and sync with cloud platforms once connectivity is restored, ensuring continuity in data collection and analysis.
Another critical aspect of accessibility is addressing the technical literacy gap among farmers. Many precision agriculture tools require a basic understanding of digital interfaces, data interpretation, and system maintenance, which can be daunting for those unfamiliar with technology. To overcome this challenge, comprehensive training programs must be implemented at the grassroots level. Agricultural extension services, in collaboration with technology providers, can offer hands-on workshops, demonstration projects, and ongoing support to help farmers build confidence in using these tools. Tailored educational materials, such as multilingual guides and video tutorials, can further simplify the learning process and cater to diverse audiences.
The design of precision agriculture tools themselves also plays a pivotal role in enhancing accessibility. Technology developers must prioritize user-centric design principles , creating intuitive interfaces and modular systems that are easy to operate and maintain. For instance, IoT sensors and drones should feature plug-and-play functionality, minimizing the need for complex setup processes. Similarly, AI-driven platforms can incorporate natural language processing to allow farmers to interact with systems through voice commands, reducing the reliance on technical expertise. By making tools more user-friendly, developers can lower the barrier to entry and encourage widespread adoption.
Furthermore, fostering collaborative ecosystems is essential to scaling accessibility. Farmers, researchers, technology providers, and policymakers must work together to co-create solutions that address real-world challenges. Open-source platforms and shared data repositories can facilitate knowledge exchange, enabling farmers to benefit from collective insights while reducing individual costs. For example, open-access satellite imagery and weather data can empower farmers to make informed decisions without needing to invest in proprietary systems. Similarly, community-driven initiatives, such as cooperative drone fleets or shared machinery pools, can enhance resource utilization and reduce financial burdens.
Finally, inclusivity must remain at the forefront of efforts to improve accessibility. Precision agriculture tools should be designed to accommodate the needs of diverse farming communities , including women, indigenous groups, and marginalized populations who often face additional barriers to adoption. Programs that provide targeted support, such as mentorship networks or gender-sensitive training modules, can ensure equitable access to these technologies. By prioritizing inclusivity, stakeholders can create a more equitable agricultural landscape where all farmers have the opportunity to thrive.
In conclusion, making precision agriculture tools accessible to all farmers requires a multifaceted approach that addresses financial, technical, and infrastructural barriers. Through innovative financing models, expanded connectivity, tailored training programs, user-friendly designs, collaborative ecosystems, and inclusive policies, we can democratize access to these transformative technologies. By doing so, we not only empower individual farmers but also contribute to a more sustainable, resilient, and equitable global food system.
How to Implement Precision Agriculture Tools: A Step-by-Step Guide
Implementing precision agriculture tools effectively requires a structured approach that ensures seamless integration into existing farming operations. Below is a detailed step-by-step guide to help farmers adopt and utilize these technologies to their fullest potential.
Step 1: Conduct a Comprehensive Needs Assessment
Before investing in any precision agriculture tool, it is essential to identify the specific challenges and goals of your farm. Conduct a thorough assessment of your current practices, resource usage, and yield performance. Key questions to consider include:
- What are the primary inefficiencies in your operations (e.g., water wastage, uneven fertilizer application)?
- Which crops or fields require the most attention?
- What are your short-term and long-term objectives (e.g., increasing yields, reducing costs, improving sustainability)?
This assessment will help you determine which tools and technologies align best with your needs. For example, if water management is a priority, IoT soil moisture sensors and automated irrigation systems may be the most suitable solutions.
Step 2: Develop a Budget and Explore Financing Options
Precision agriculture tools often involve significant upfront costs, so it is crucial to establish a realistic budget. Consider both the initial investment and ongoing expenses, such as maintenance, software subscriptions, and training. Explore financing options to make these tools more affordable:
- Apply for government subsidies or grants aimed at promoting sustainable farming practices.
- Partner with other farmers to share the cost of expensive equipment like drones or GPS-guided machinery.
- Negotiate leasing agreements with technology providers to spread out payments over time.
Step 3: Select the Right Tools and Technologies
Based on your needs assessment and budget, choose the precision agriculture tools that best address your challenges. Some key considerations include:
- GPS-Guided Machinery: Ideal for optimizing field operations and reducing overlap.
- Drones: Excellent for crop monitoring, pest detection, and field mapping.
- IoT Sensors: Perfect for real-time monitoring of soil moisture, temperature, and nutrient levels.
- AI Platforms: Useful for predictive analytics, decision support, and resource optimization.
Ensure that the selected tools are compatible with each other and can integrate into a cohesive system. For instance, IoT sensors should be able to transmit data to cloud-based platforms that analyze and visualize the information.
Step 4: Invest in Training and Capacity Building
To maximize the benefits of precision agriculture tools, farmers and farmworkers must be adequately trained to use them. Collaborate with technology providers, agricultural extension services, or educational institutions to organize training programs. Key areas to focus on include:
- Operating and maintaining the tools (e.g., calibrating sensors, programming drones).
- Interpreting data generated by the tools (e.g., analyzing NDVI maps, understanding soil moisture reports).
- Integrating the tools into daily farming activities (e.g., adjusting irrigation schedules based on sensor data).
Hands-on workshops and practical demonstrations are particularly effective in building confidence and competence.
Step 5: Pilot Test the Tools on a Small Scale
Before fully integrating precision agriculture tools into your operations, conduct a pilot test on a small section of your farm. This allows you to evaluate the tools' performance, identify potential issues, and make necessary adjustments. For example, if you are testing an IoT-based irrigation system, monitor its impact on water usage and crop health over a growing season. Use the insights gained from the pilot test to refine your approach before scaling up.
Step 6: Integrate Data Collection and Analysis Systems
Precision agriculture relies heavily on data, so it is essential to establish robust systems for collecting, storing, and analyzing information. Invest in cloud-based platforms that aggregate data from multiple sources, such as IoT sensors, drones, and satellite imagery. These platforms should offer advanced analytics capabilities, such as generating vegetation indices, predicting pest outbreaks, and recommending optimal interventions. Ensure that the data is easily accessible and actionable, allowing you to make informed decisions quickly.
Step 7: Monitor and Optimize Performance
Once the tools are fully integrated, continuously monitor their performance and impact on your farm. Track key metrics such as yield improvements, resource savings, and cost reductions. Regularly review the data generated by the tools to identify trends and areas for further optimization. For example, if soil moisture sensors indicate consistent over-irrigation in certain zones, adjust your irrigation strategy accordingly.
Step 8: Scale Up Gradually
After achieving success on a small scale, gradually expand the use of precision agriculture tools across your entire farm. Start with high-priority areas, such as fields with the greatest potential for improvement, and then extend to other sections. Scaling up incrementally allows you to manage risks and ensure that the tools deliver consistent results.
Step 9: Foster Collaboration and Knowledge Sharing
Engage with other farmers, industry experts, and technology providers to stay updated on the latest advancements in precision agriculture. Participate in forums, conferences, and online communities to share experiences and learn from others. Collaborative efforts, such as forming cooperatives or joining regional farming networks, can also enhance resource sharing and collective problem-solving.
Step 10: Evaluate Long-Term Impact and Adapt
Finally, periodically evaluate the long-term impact of precision agriculture tools on your farm's productivity, profitability, and sustainability. Assess whether the tools are meeting your initial goals and identify opportunities for further improvement. Be prepared to adapt your strategies as new technologies emerge and market conditions evolve.
By following this step-by-step guide, farmers can successfully implement precision agriculture tools, unlocking their full potential to drive efficiency, sustainability, and resilience in farming operations.
Conclusion: The Transformative Power of Precision Agriculture Tools
Precision agriculture tools represent a paradigm shift in the way farming is conducted, offering unparalleled opportunities to enhance productivity, sustainability, and resilience. By leveraging advanced technologies such as GPS-guided machinery, drones, IoT sensors, and AI-driven platforms, farmers can transition from generalized practices to highly customized and data-centric approaches. These tools enable precise monitoring and management of resources, early detection of crop stressors, and optimized decision-making, ultimately leading to higher yields, reduced input costs, and minimized environmental impact.
The adoption of precision agriculture tools is not merely a technological upgrade but a strategic investment in the future of farming. As global challenges such as climate change, population growth, and resource scarcity intensify, the ability to produce more with less becomes increasingly critical. Precision agriculture provides a viable solution by maximizing resource efficiency and promoting sustainable practices. For instance, variable rate technology reduces fertilizer overuse, while IoT-enabled irrigation systems conserve water—both of which contribute to a smaller ecological footprint.
Moreover, the scalability and adaptability of precision agriculture tools make them accessible to farms of all sizes, from smallholder operations to large commercial enterprises. Modular designs, shared resource models, and innovative financing options ensure that even resource-constrained farmers can participate in this digital agricultural revolution. By democratizing access to advanced technologies, precision agriculture fosters inclusivity and equity, empowering diverse farming communities to thrive.
Looking ahead, the continued evolution of emerging technologies promises to further amplify the impact of precision agriculture. Innovations such as blockchain, 5G connectivity, autonomous robots, and biotechnology are set to redefine the boundaries of what is possible in farming. These advancements will enable even greater levels of precision, automation, and predictive capability, driving efficiency and sustainability to new heights.
In conclusion, precision agriculture tools are not just instruments of modern farming—they are catalysts for transformation. By embracing these technologies, farmers can position themselves at the forefront of a new era in agriculture, marked by unprecedented levels of productivity, sustainability, and resilience. The journey toward smarter, more efficient farming practices begins with the adoption of precision agriculture tools, paving the way for a brighter and more sustainable future for generations to come.
10 Frequently Asked Questions (FAQs) About Precision Agriculture Tools
-
What are precision agriculture tools?
Precision agriculture tools are advanced technologies designed to optimize farming practices by providing accurate data and enabling precise control over resources such as water, fertilizers, and pesticides. Examples include GPS-guided machinery, drones, IoT sensors, and AI-driven platforms. -
How do precision agriculture tools improve efficiency?
These tools enhance efficiency by enabling farmers to allocate resources with pinpoint accuracy, reducing waste, and ensuring that inputs contribute directly to crop health and yield. For instance, variable rate technology adjusts fertilizer application based on soil nutrient profiles. -
Are precision agriculture tools suitable for small-scale farmers?
Yes, many precision agriculture tools are scalable and can be adapted for small-scale operations. Shared resource models, such as cooperative drone fleets or IoT sensor networks, make these technologies accessible to smallholder farmers. -
What is the role of drones in precision agriculture?
Drones equipped with multispectral cameras and thermal sensors provide aerial insights into crop health, detect pest infestations, and generate detailed field maps. They enable early detection of stressors and support targeted interventions. -
How do IoT sensors benefit farming?
IoT sensors monitor real-time environmental parameters such as soil moisture, temperature, and nutrient levels. This data helps farmers optimize irrigation schedules, apply fertilizers precisely, and detect early signs of crop stress. -
Can precision agriculture tools reduce environmental impact?
Yes, these tools minimize the over-application of chemicals and water, reducing runoff, leaching, and greenhouse gas emissions. They promote sustainable farming practices by optimizing resource usage and protecting soil health. -
What are the costs associated with adopting precision agriculture tools?
Costs vary depending on the tools and scale of implementation. While initial investments can be high, financing options such as subsidies, leasing programs, and shared ownership models can make these technologies more affordable. -
Do I need technical expertise to use precision agriculture tools?
Basic technical knowledge is beneficial, but many tools are designed with user-friendly interfaces. Training programs and support from technology providers can help farmers build the necessary skills. -
How do AI and machine learning enhance precision agriculture?
AI and machine learning analyze vast datasets to predict trends, recommend actions, and optimize resource allocation. For example, predictive models forecast pest outbreaks, enabling preemptive measures. -
What are the future trends in precision agriculture?
Emerging trends include blockchain for supply chain transparency, 5G connectivity for real-time data transmission, autonomous robots for labor-intensive tasks, and biotechnology for developing resilient crop varieties.
What's Your Reaction?
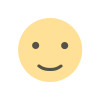
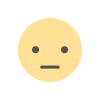
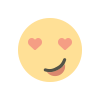
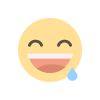
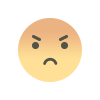
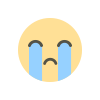
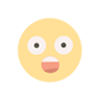